Beyond 120
Ideas from the father of modern stem cell biology, Irving Weissman, are starting to blossom into a future where we get old slower—and live longer
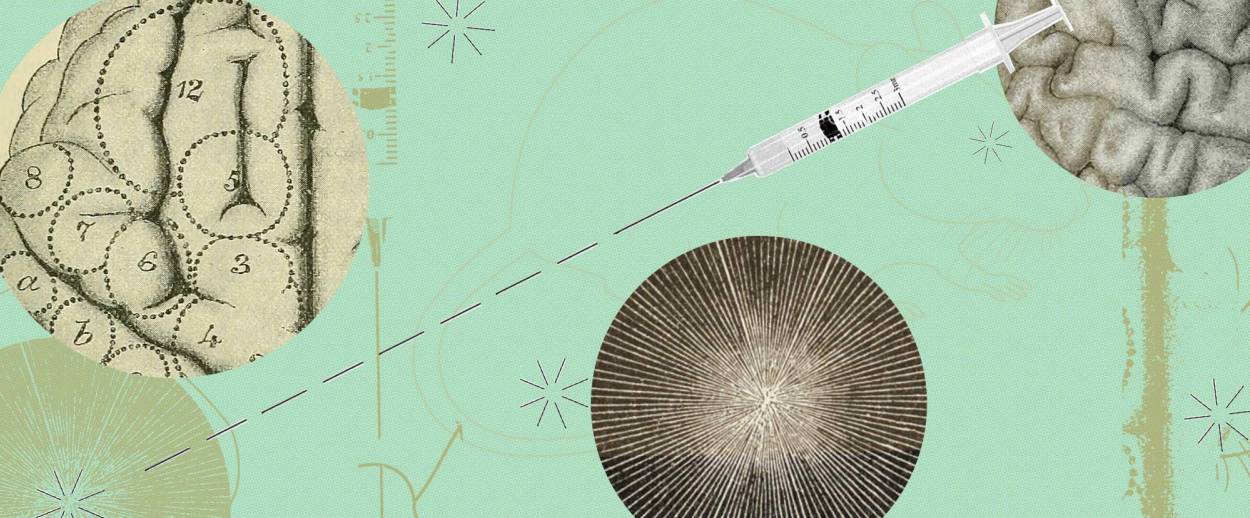
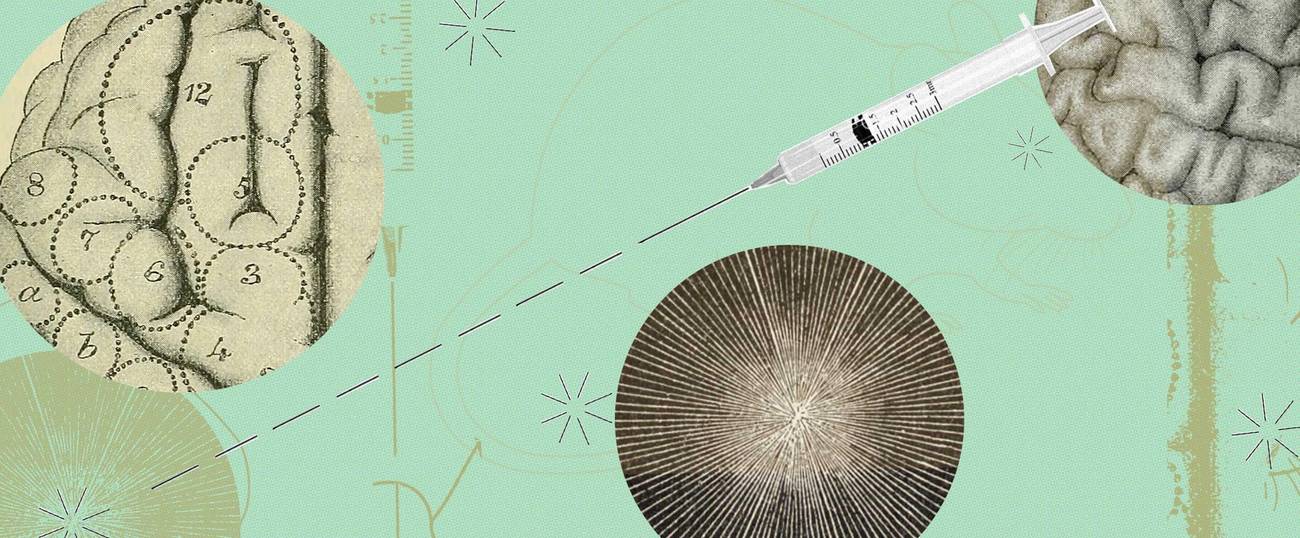
Plato tells a story, familiar to most of us, of how tools and abilities were distributed to all the animals, fangs and claws and stingers, yet all of these gifts were gone when mankind reached the head of the line. Prometheus stole fire from Hephaestus and technology from Athena, but that wasn’t enough for mankind to survive. Realizing this, Plato tells us in Gorgias, Prometheus gave us another, a much greater gift. We’ll get to that in a moment.
First, let’s go over what will happen to us as we grow old. Sometime after age 50, depending on personal genetics and life history, our gums withdraw, we lose our hair, our saliva glands falter, and our teeth grow brittle and break off or fall out. Our skin gets thinner, less flexible; it sags, wrinkles, and is discolored by “liver spots.” Our bones lose density and strength and shrink in size as our joints swell. Our shoulders slump, our spines buckle and hump. Our muscles atrophy and waste away so we lose mobility as we grow progressively weaker. Our balance and hearing deteriorate. Our eyes dry and lose their ability to focus, so we’re more likely to fall, and our bones break more easily. We’re slower to heal and more vulnerable to infection as we do, if we do. Hormone levels change. Our memory fails, and most of us, almost all of us, will develop dementia if we live long enough. We’ve reached the golden years; taking away foreknowledge of them was Prometheus’ greatest gift to mankind.
Living until 120, the life-span traditionally attributed to Moses, seems more like a curse than a blessing. But it doesn’t have to be that way. I’ve spent the last year talking with scientists around the world about why we’ve been so successful treating the diseases of youth and middle age and yet haven’t made similar progress against end-of-life afflictions. What I found is that scientists at Stanford, Harvard, USC, Wake Forest, UC Berkeley, San Francisco, USC, and Cambridge University, at Scripps Institute, the SENS Research Foundation, and Buck Institute for Research on Aging are unanimous in agreement: Science has gained the ability to intervene successfully in the aging process and to delay and to selectively reverse its effects. The speed at which these new technologies and techniques—which now exist—move from the lab to the clinic is directly dependent on public awareness and support.
***
In 1907, average life expectancy in the United States was 45.6 years. In 2013, according to the World Health Organization, it was 79. Yet that huge jump mostly reflects the tremendous success we’ve had detecting and treating infectious diseases that used to take us out as infants, children, and young adults. As a result of this success, most of us can now expect to live into old age, which means that more of us than at any previous time in history can expect to suffer from the multitude of opportunistic afflictions we can’t cure and that require care that even an advanced society like ours can’t hope to pay for. “Increased disability rates are now accompanying increases in life expectancy, leaving length of a healthy life span unchanged or even shorter than in the past,” says Dana Goldman, professor of Public Policy and Pharmaceutical Economics at USC. While we are less likely to fall victim to a single disease as we age, multiple competing causes of death directly associated with biological aging are likely to carry us off.
Alzheimer’s disease is the best-known degenerative brain disease of old age. For people at age 65, according to the Centers for Disease Control and Prevention, the risk of developing the disease begins to double every five years. By age 85 years and older, between 25 percent and 50 percent of people will exhibit signs of Alzheimer’s disease. Most of us will live four-eight years after diagnosis; some of us for 20 years or more. About 40 percent of our lives after Alzheimer’s diagnosis will be spent in the most severe stage of the disease. So hideous is the affliction and so ineffective are existing treatments that physicians debate whether it is acceptable to keep a diagnosis from the patient.
Brock Reeve, director of the Harvard Stem Cell Institute, says, “Alzheimer’s is a ticking time bomb for society. It’s going to totally shoot the healthcare economics of this country.” In 2015, America’s total payments for healthcare, long-term care, and hospice for people with Alzheimer’s and other age-related dementia are estimated to be $226 billion. Medicare: $113 billion; Medicaid is $41 billion; insurance deductibles and out-of-pocket payments: $44 billion; and $29 billion “other,” meaning payment sources including private insurance, health maintenance organizations, managed-care groups, and uncompensated care. When what health care professionals call “the silver tsunami” hits, healthcare costs will increase astronomically. By 2050, 88.5 million Americans will be over 65, and estimated costs for Alzheimer’s care alone will run from $1 trillion and upwards. As USC’s Dana Goldman explains, “Medicare spending alone is projected to double as a share of gross domestic product, from 3.7 percent in 2012 to 7.3 percent in 2050.” The number of treatments to slow or stop the relentless progression of Alzheimer’s disease now available is zero.
One response to this building crisis was the 2011 “National Alzheimer’s Plan” (NAPA), which starts with the mandatory “vision statement”:
For millions of Americans, the heartbreak of watching a loved one struggle with Alzheimer’s disease is a pain they know all too well. Alzheimer’s disease burdens an increasing number of our nation’s elders and their families, and it is essential that we confront the challenge it poses to our public health. —President Barack Obama
The plan runs on through turgid pages of acronyms and good ol’ can-do, roll-up-the-sleeves huff ‘n’ puff that reads like a 1950s horror film script. The NAPA plan concludes, optimistically, on page 31,with Goal5, Strategy 5B: Monitor progress on the plan.
There hasn’t been any.
Irving Weissman M.D., Ludwig professor for Clinical Investigation of Cancer research at Stanford and director of the Institute for Stem Cell Biology and Regenerative Medicine there says, “We have a perfect storm at the NIH now, sadly, in that mediocre people get to judge whether the experiments will work, and so, as money has fallen back … it becomes more and more critical that the money that is spent should go to the most adept experimenters.” Weissman, considered by many scientists to be the father of modern stem-cell biology, says that what’s happening now, with the NAPA plan, “is just the opposite.”
A molecular neurobiologist less senior than Weissman and more vulnerable to retaliation at this stage of his career told me bluntly in a recent email that the billions spent on decades of Alzheimer’s research “wasn’t spent on funding a large basket of ideas and looking under every rock” because “NIH is in the habit of funding the same old tired ideas from the usual suspects.” The same scientist added that the National Alzheimer’s Plan is “run by the same group of NIH-connected people who have been running it (right off a cliff) for the last decade.” This neurobiologist, who did post-doctoral work at the Max Planck Institute for Psychiatry in Germany, also noted that the plan now limits “who can apply for grants to a ‘pre-screened pool’ of investigators.” These are the same ones characterized as “mediocre,” by the father of modern stem-cell biology, Irving Weissman.
***
Scientists have traditionally studied diseases separately because they have separate pathologies. Heart disease mostly comes from accumulated fat deposits clogging arteries, cancers from DNA damage, Alzheimer’s and other dementias from damaged brain cells, etc.—and each disease has multiple contributing factors. But they share a common feature: Aging drives them all. If we delay aging and rejuvenate organs, tissues, and cells, we can prevent or remediate them all. According to Nir Barzilai, director of the Institute of Aging Research at Albert Einstein College of Medicine, “Although aging is the major risk factor for developing most adult-onset diseases, systematic investigations into the fundamental physiology, biology, and genetics of aging are only just beginning.”
There’s good reason to be confident that moving away from the “infectious disease” model and shifting research dollars from individual diseases of aging to the basic biology of aging will be productive. Not only because such a shift upgrades the theoretical model on which research is based, but because it already has been. A seminal paper in this field was published in Nature in 2005. “Rejuvenation of aged progenitor cells by exposure to a young systemic environment” had profound implications and initiated multiple lines of investigation being actively pursued by the scientists involved in the original experiments and by many others in labs at universities, research institutions, and pharmaceutical companies around the world.
Irina Conboy, the paper’s lead author and now an associate professor in U.C. Berkeley’s Department of Bioengineering, told me that the idea for the experiment originated with her husband, Michael Conboy. At the time both Conboys were working as senior scientists, postdoctoral researchers in Thomas Rando’s Stanford lab.
Michael Conboy, now also a professor at Berkeley, told me, “We [Irina and I] had been discussing at home over dinner how all the organs and tissues in the body age together; they all go to hell in a hand basket at the same time … whether they’re highly metabolic tissues like skin, which is turned over frequently, or something that’s never turned over, like differentiated neurons.” Conboy added, “That suggested there was something in common that coordinates or facilitates this aging decline.”
Irina Conboy wanted to confirm her husband’s conjecture that pro-aging and pro-rejuvenation factors exist in the blood of old and young animals using a surgical technique called “parabiosis” (para, beside, biosis, living) to join the circulatory systems of two mice. Since in this proposed experiment the mice sharing their common blood supply would be of different ages to allow scientists to study the effect of exposure to one another’s blood, it would be called “heterochronic parabiosis (hetero, different, chronic, ages).
Today, before a researcher can conduct an experiment using animals that may or must die, he or she must submit a written protocol describing the proposed experiment to an institutional committee for review. In order to be approved the protocol document must include a comprehensive description of anesthetic, analgesic, and surgical methodology and postsurgical care. It must include a historical review of the procedure and the scientific rationale that necessitates it, as well as evidence of the surgeon’s training, prior experience, and skill. In the case of parabiotic surgery, where the mortality is high, the requirements for approval are particularly demanding.
Irving Weissman is experienced with parabiotic surgery. He learned how to perform the delicate operation while still in high school, working in the lab of Ernst J. Eichwald, a scientist who fled the distractions of academic research to work as a pathologist in a hospital in Great Falls, Montana, where he set up a lab to study the genetics of transplant rejection. As a result of his early apprenticeship, Weissman was lead author on his first scientific paper, published in 1962, the same year he started medical school at Stanford.
Weissman, now 75, is still incredibly productive; his Stanford lab’s most recent contribution to science is a breakthrough treatment for cancer, “an antibody therapy to cancer stem cells that treats all human cancers, not just leukemia.” When Irina Conboy approached Weissman about performing the heterochronic parabiosis experiment, she recalls, Weismann said yes “before I could finish the sentence.”
Blood is data soup, a rich broth of encoded information that dynamically orchestrates the interaction of every cell and organ system in the body, an intricate liquid network of great beauty and enormous complexity, a continuously flowing, ever changing, interacting, interdependent skein of looping metabolic pathways throughout the body.
Weissman and his then-postdoctoral student Amy Wagers (now at Harvard, with her own lab) joined the Conboys, Tom Rando, and neurobiologist Eric Girma to carry out the experiment in Rando’s Stanford lab. Old and young mice were surgically joined at the flank so they shared blood. After two months they were sacrificed, and their organs (including liver) and muscle tissue were examined at the molecular and genetic levels, using techniques and technology unavailable just a few years ago.
Although the scientists hoped to trace changes in some areas, they were by no means expecting to see the pronounced and dramatic effects that they saw. The results of the meticulous experiment demonstrated that exposure to young blood’s “systemic environment” revitalized “aged progenitor cells” (akin to stem cells, but more specific), confirming the hypothesis “that there are systemic factors that support robust regeneration of tissues in young animals and/or inhibit regeneration in old animals, and that these factors act to modulate key molecular pathways that control the regenerative properties of progenitor cells.” In other words, the young blood serum restored aging progenitor/stem cells to youthful levels of functionality. The result, the Rando-Weissman joint lab’s paper reported, was the rejuvenation or regeneration of both muscle and liver tissue. The scientists measured, charted, took micrographs, and comprehensively documented the rejuvenation effects on muscle and liver tissue in their published paper.
What the Rando-Weissman lab team documented and published in the report was dramatic enough even in understated scientific prose: “The decline of tissue regenerative potential with age can be reversed through the modulation of systemic factors.” Yet there was more cause for excitement that wasn’t in the published paper.
The Rando Lab’s researchers also saw evidence of rejuvenation and increased cell proliferation in other, unexpected, areas of the body, including the heart and the brain. Mature neurons in brains, like most cardiac cells, are considered “postmitotic”: They rarely divide, and no one expected to see new neurons or cardiac cells—but they did. If it was possible to rejuvenate old brains sufficiently to improve memory and cognition by growing new functional neurons, you could treat people now helpless with Alzheimer’s disease and other dementias, and you could also treat people early to prevent them ever getting those diseases. Likewise, if new functional cardiac cells were really being created you could treat the most common heart-related cause of death, age-related heart failure, which, like Alzheimer’s, is currently untreatable.
***
Blood stirs things up with us; it’s a potent trigger for emotions of all kinds, an archetypal symbol that inevitably generates powerful feelings and impulses rooted in archaic brain structures beyond most people’s conscious control. It is an organ that connects almost every cell in the body; it carries nutrients and metabolic waste, oxygen, and carbon dioxide to and from our lungs. It is the transport medium for our immune system, an interlocking complex of signaling proteins and cellular machines like white blood cells and phagocytes—micro machines that engulf and disassemble bacteria and dead cells and that learn, remember, and protect us from disease. The plasma fraction of blood carries vast quantities of information encoded in thousands of proteins; enzymes, hormones, blood factors, and signaling molecules like exosomes and plasmids. Blood is data soup, a rich broth of encoded information that dynamically orchestrates the interaction of every cell and organ system in the body, an intricate liquid network of great beauty and enormous complexity, a continuously flowing, ever changing, interacting, interdependent skein of looping metabolic pathways throughout the body.
Learning to decode, understand, copy, and to send those signals encoded in blood factors or other macromolecules to old hearts, brains, and muscles, to instruct organ systems to rejuvenate, to repair themselves, is the first step of the initial phase of discovery. All protein signals in blood can ultimately be decoded, which will allow us to exercise precise targeted control over all manner of biological activity. The scientific equipment to do this is increasingly available and increasingly automated. The “magic” of young blood is contained in the instructions encoded in circulating proteins, which can be synthesized inexpensively.
In the wake of the 2005 publication of “Rejuvenation of aged progenitor cells” in Nature, scientists at Stanford, Harvard, UCSF, University of Maryland, and at many other research institutions, foundations, and pharmaceutical companies around the world have been scrambling to identify the factors in blood plasma that are responsible for rejuvenation as well as the “pro aging” factors that are implicated in biological decline. Amy Wagers, now professor of Stem Cell and Regenerative Biology at Harvard Stem Cell Institute, working with Richard Lee, a Harvard Medical School professor and a cardiologist at Brigham and Women’s Hospital, has identified a protein, GDF11, found in young plasma and largely absent in old. GDF11 has proved to be sufficient when injected to reverse signs of aging in the hearts of old mice.
In another experiment, Wagers, working with Lee Rubin, also at Harvard Stem Cell Institute, showed that GDF11 has a similar rejuvenative effect on muscles and brains of old mice. Wagers emphasizes the significance of these findings in a 2014 story available on the HSCI web site: “Prior studies of young blood factors have shown that we achieve restoration of muscle stem cell function, and they repair the muscle better. In this study, we also saw repair of DNA damage associated with aging, and we got it in association with recovery of function, and we saw improvements in un-manipulated muscle.” When I asked Wagers how long the effects persisted, she told me, “In muscle […] you can still see improved regeneration 3 weeks later, but that is as far as we have tested it.”
Some of Wagers’ results are questioned by other scientists, including Irina Conboy. Thomas Rando, in whose Stanford lab the 2005 experiment was carried out, is acquainted with both parties and has detailed knowledge of the experiments and results. He says, “I bet they’re both right.”
What Rando meant was when work done by researchers of the caliber of the Conboys and Wagers show discrepancies like this, such disagreements are almost invariably found to be the result of unrecognized differences in methodology, definition, or unreliable assay tools. But there is no doubt about the reality of the rejuvenative process they’re observing.
Tony Wyss-Coray, of Stanford, heard from Rando about the neurogenesis that was seen but not documented or published in the 2005 experiment. Wyss-Coray, who had long studied Alzheimer’s disease, decided to do an experiment using heterochronic parabiosis, but with the intention of studying first the effect of old blood not on the liver or muscle, but on the brains of young mice.
Saul Villeda had just returned from a year off to complete his PhD working in Wyss-Coray’s Stanford lab. Villeda recalls Wyss-Coray wondering whether the blood factors, like those he’d seen in his studies of Alzheimer’s patients, were biomarkers of aging, or whether some of them might actually communicate, or possibly even control switches in the DNA or other software involved in cell functions.
The Wyss-Coray lab team decided to use heterochronic parabiosis to look at the effect of old mouse plasma on young mice at the peak of their cognitive abilities. What they found was that “exposing a young mouse to an old systemic environment or to plasma from old mice, decreased synaptic plasticity, and impaired contextual fear conditioning and spatial learning and memory.” In other words, the young mice treated with old blood were strongly adversely affected by pro-aging factors found in old blood. There are a number of tests to measure mouse intelligence, and some of these showed that young mice exposed to old plasma had dramatic declines in cognitive ability. The treated mice were “really pretty stupid,” says Villeda.
Having shown that old mouse plasma adversely affected cognition, the next question was whether young mouse plasma would improve cognitive capacity in old mice. The Wyss-Coray lab team decided to do another parabiosis experiment to find out. Villeda says now that he didn’t “really think it would work.” Changing cell mechanics was one thing, but the idea of actually improving cognition in a mouse that’s been around 18 months? “That’s the equivalent of a person in their 60s,” admitted Villeda. “That seemed crazy.”
Yet the Wyss-Coray lab went ahead and established that significant cognitive improvements and neurogenesis had both occurred. But when they submitted their findings to Nature in 2012,the authoritative scientific publication balked. Nature’s editors wanted more data; they wanted to know what, exactly, was going on in the brain that might account for the cognitive improvements the Wyss-Coray lab had seen before they would publish such unprecedented results. Was such a thing even remotely possible? The implications were profound if it was.
When Villeda got his own lab at UCSF in 2012, he and Wyss-Coray decided to repeat the experiment there. Villeda had his graduate students at UCSF conduct a blinded experiment independent of him. When they got the same results, found the same cognitive improvements as seen in the earlier Stanford experiment, they also did genome-wide micro-array analysis of the hippocampus region (the brain area involved in learning and memory formation) and established that “exposure of an aged animal to young blood can counteract and reverse pre-existing effects of brain aging at the molecular, structural, functional and cognitive level.”
There was no longer any doubt. When the lab resubmitted their paper to Nature Medicine in February 2014, it was accepted. In May of that year “Young blood reverses age-related impairments in cognitive function and synaptic plasticity in mice,” was published in Nature Medicine. Wyss-Coray and Villeda were delighted. They’d seen cognitive improvement and structural regeneration at Stanford, and now, again, at UCSF.
***
Mice share just 92 percent of their genome with humans, have a higher metabolic rate, and can you say “eek!” Yet they also have the same organs and systemic physiology as monkeys and human beings do and use similar mechanisms to regulate growth, replication, and cell differentiation. Those are the metabolic processes involved in rejuvenation and regeneration of age-damaged tissues and organs.
To roughly assess the approximate similarity of the communication mechanisms between species of mice and men, Joseph Michael Castellano, a young researcher in Wyss-Coray’s lab, gave young human plasma to old mice. Castellano was prohibited from discussing his data prior to publication, but Wyss-Coray told me and repeated in his talk at the SENS Foundation’s Rejuvenation Biotech Conference in San Francisco that they had seen young human plasma produce the same rejuvenative effect in mice as young mouse plasma had.
All biological networks, man-made or not, must reliably transmit information. When networks can’t do this, organisms that use those networks to coordinate their functions fail.
Wyss-Coray is a neurologist; he’s studied Alzheimer’s disease and is intimately familiar with the relentless, devastating progression of the pathology of the disease, the futility of existing treatments, and the emotional and financial suffering and exhaustion of many of the families affected. He decided that a clinical trial using young human plasma to treat Alzheimer’s disease in human beings was warranted.
Organizing a clinical trial to treat old people with mental impairments is not easy. The patients must be recruited; caregivers, family members, or both, must be willing to accompany patients to Stanford and care for them for the duration of an 18-week trial. Wyss-Coray told me in an email at the beginning of the year that the first clinical trial ultimately recruited too few patients to produce publishable data. What the Stanford trial team did see in the few patients treated, while insufficient for publication, was enough to justify starting a new, expanded clinical trial immediately. He expects to have publishable data by summer of 2016.
***
Alzheimer’s is just one tiny slice of the much larger puzzle of aging. Scientists all along the ragged frontier of our advancing biological knowledge are discovering and doing things inconceivable 10 years ago.
At the Scripps Research Institute in La Jolla, Jeanne Loring, a researcher with dual competences in stem-cell biology and genetics, reprograms cells from patients with Parkinson’s disease and turns them by stages into dopamine-producing neurons, the brain cells Parkinson’s sufferers lack. Loring and her clinical partner Melissa Houser M.D., director of Parkinson’s Disease and Movement Disorder Center at Scripps Clinic, are applying to the FDA for permission to implant the cells in Parkinson’s patients, a process that Loring estimates will take two to three years. She is optimistic about the possibility of success because “a similar procedure was successfully done in the 1990s using fetal tissue, and now, about 20 years later, some of those people have died and their brains examined; the cells that were transplanted were perfectly healthy and the patients had had no Parkinson’s disease symptoms for the rest of their lives.”
Anthony Atala, M.D., a surgeon and the director of the Wake Forest Institute for Regenerative Medicine, and Chair of the Department of Urology at Wake Forest University, focuses on growing new human cells, tissues, and organs. Atala’s team at Wake Forest has created functional bladders and implanted them in patients, has done the same with penises and vaginas, and, using a modified ink-jet printer to spray stem cells on a collagen lattice, has made functional kidneys as well.
Irina and Michael Conboy, working with David Schaffer, director of the Berkeley Stem Cell Center and a professor of chemical and bio-molecular engineering, showed, in a paper published in 2015, that interrupting the function of TGF-β1 (Transforming Growth Factor Beta 1), which is elevated by aging, makes that pathway “behave younger” and “rejuvenates multiple organ systems.” This finding suggests that at least some part of the rejuvenative process is activated by removing, de-activating, or inhibiting TGF-β1.
These findings are so surprising it’s tempting to overlook other less dramatic, equally important work being done in creating a more complete understanding of the basic biology of aging. Judy Campisi at the Buck Institute for Research on Aging has made multiple fundamental contributions to our understanding of the biology of aging. One recent finding of significance is that Rapamycin, beneficial to aging brains (suppressing harmful secretions from senescent cells), can effectively do this with intermittent dosing.
***
If you think this progress seems to be happening very quickly, you’re correct, and there’s a reason why this is so. George Church, professor of Genetics at Harvard Medical School and Professor of Health Sciences and Technology at Harvard and MIT and one of the world’s foremost geneticists, wrote in a recent forward to a lab manual on synthetic biology, “The elephant (or mammoth) in the room—’Exponential Technologies.’ The rate of change in biotechnologies (about eightfold a year) has exceeded even the super rate of Moore’s Law for electronics. … DNA sequencing was going about 1.5-fold per year from its start in 1968 until 2005, when ‘next generation sequencing’ began.” When I asked Church about the effect of that change in biorejuvenation technologies, he told me, “We’re no longer limited to small-scale experiments; even small labs can do large scale experiments using next gen sequencing […] rejuvenation builds on, or drafts on, that progress.”
George Church is a geneticist, and genetics greatly benefited from the integration of computational technology into research. Now every scientist I talked to told me the same thing was happening in his or her specific field. Not only has the integration of computer technology into biological research allowed us to see and do things impossible before, it allows us to do them faster and more accurately and to use those results to improve, accelerate, or optimize the research process itself. Research that would have been impossible just a few years ago can now be done in a year; next year, such work will be done in months.
We don’t intuitively grasp exponential rates of change. It’s hard for us to handle and examine them with our imagination because we have little or no experience of them in daily life. Exponential growth in knowledge creation is what’s happening, and this is not merely new knowledge being created faster than before.
Yet knowledge is fundamentally different from a thing, like an iPhone; as the product of a creative process, according to the quantum computational physicist David Deutsch, who has written of this extensively, it is unpredictable in principal. We can’t know what we will discover, nor therefore can we anticipate the dangers that powerful new technologies will present.
As computational techniques and technologies become more deeply integrated into all the sciences, as Deutsch predicts, what we are currently seeing is a rapid change in the rate of creation of new, objectively true knowledge. Deutsch also points out, as a matter of fact, not speculative opinion, that at some point an increasing rate of change of knowledge creation must turn exponential. That’s a physical law. If our knowledge creation processes aren’t disrupted by rising social disorder, increasing political polarization, economic disaster, or any other reason, then they must transform our world more dramatically, rapidly, and irreversibly than internal combustion engines, electricity, the transistor, and nuclear energy have already done.
Yet our existing social institutions and our legal and regulatory structures date in many cases to the 1700s and before. Legal, political, and economic structures were intended to accommodate a much slower, arithmetical, additive, rate of change. The absence of trained reporters at the science desks of major networks and news organizations is also huge problem and a direct consequence of the decay of our traditional news outlets, which were rooted in 16th-century technology and flattened by the digital age.
Thanks to vast increases in computational power, we have the ability to reverse aging and cure diseases that otherwise threaten our economic and social order. Yet our regulatory and political systems prevent new technologies from ever reaching us, and the decay of our traditional information gathering and distribution systems means we never hear about them and never know what we, as a species, are now capable of. The future is not delivered to us, we must choose it, said Erwin Schrodinger; but before we do we must know what is available to us.
We can accommodate change by using the same technologies that now bedevil us by taking what we learn from the study of biological networks and using it to improve and error correct the networks we create.
All biological networks, man-made or not, must reliably transmit information. When networks can’t do this, organisms that use those networks to coordinate their functions fail. Social organisms are organisms and rely on networks to distribute information critical to their function. The more technologically advanced a society is, the more dependent on its information supply. The quality of information available becomes the chief limiting factor in the development of civilization.
Securing our networks, understanding that that information quality and availability is essential to collective social function, will allow us to manage our future, reorganize our understanding, and access more knowledge in less time than ever possible before.
Not securing our networks means civilization will falter, collapse, and die.
***
Ted Mann last wrote for the magazine about Kinky Friedman.
Ted Mann is an Emmy award-winning writer who worked on NYPD Blue, Deadwood, Hatfield McCoy, and Homeland.